Fiber Optic Sensing Technologies for Bridge Health Monitoring
✅ Paper Type: Free Essay | ✅ Subject: Technology |
✅ Wordcount: 2964 words | ✅ Published: 23 Sep 2019 |
A Review of Fiber Optic Sensing Technologies for Bridge Health Monitoring
Abstract
The application of structural health monitoring (SHM) systems in bridge infrastructures has lead to the enhanced safety, durability, reliability and cost-effective management of these structures. In the last two decades, a significant number of innovative sensing technologies based on optical fiber sensors have been applied for SHM due to their inherent distinctive advantages, such as small size, light weight, immunity to electromagnetic interference (EMI) and corrosion, and embedding capability. Fiber optic-based monitoring systems use quasi-distributed and continuously distributed sensing techniques for real time measurement and long term assessment of physical and engineering properties of bridges. This allows for early stage damage detection and characterization, leading to timely remediation and prevention of catastrophic failures. The purpose of this article is to review the current state-of-the-art of fiber optic sensing/monitoring technologies, including the basic principles of various optical fiber sensors, novel sensing and computational methodologies, and the practical application status of this technology for bridge health monitoring. Additionally, application of these technologies to monitor temperature, inclination, stresses, strain measurements, vehicle speeds and weights, and corrosion and cracking of reinforced and prestressed concrete structures of bridges will also be discussed.
1. Introduction
Bridges play a vital role in advancing nation’s transport infrastructure. Loss of lives and financial losses have been caused by bridge failures (Li, Ou, and Zhou 2009). Bridges deteriorate with age and early detection of damage helps in timely remediation preventing catastrophic failures. Several bridges built decades ago are now subjected to various load patterns and environmental changes, which can cause localized distress and if not corrected can result in bridge failure. Therfore, structural health monitoring is necessary to ensure bridge safety. SHM refers to the procedure used to assess the condition of structures over a period of time using appropriate sensors to monitor their performance, and measurements yeilded by these sensors can help in early damage detection and risk assessment, thus increasing reliability, safety and efficiency of the structures.
Recent advancements in fiber optic sensing (FOS) techniques offer unique advantages for i) long-term monitoring, ii) large distances (in the range of several kilometers), and iii) early detection of risks associated with damage of civil infrastructures. One of the distinct advantages of FOS is their ability to measure physical quantities (such as displacements, inclination and strains) continuously distributed over the full length of the fiber. In order to measure distributed strains over the full
length of a fiber, several techniques have been proposed, including Fiber Bragg Gratings (FBG), scattering based distributed sensors following the principle of optical reflectometry and interferometry (Fabry-Perot (FP)) based sensors. These sensing systems are easy to install, capable of autonomous operation, and are designed to measure strains and other physical parameters induced due to mechanical and thermal stresses. The objective of this paper is to review the current state-of-the-art of FOS technologies and its application for monitoring bridge infrastructures and its associated components.
2. Fiber Optic Sensing Technologies
A fiber optic sensing system consists of a light transmitter, a receiver, an optical fiber, a modulator element, and a signal processing unit. These systems are based on the variations of physical characteristics of optical fibers such as light intensity, frequency/wavelength, phase, and polarization, which are sensitive to various external signals/stimuli. Based on the sensing principle, fiber optic sensors can be categorized into different types as illustrated in the following sections.
2.1 Fiber Bragg Grating (FBG) Sensors
FBG sensors consist of varied refractive indices in the core of optical fiber (Peters 2009). According to Bragg’s law, a beam of white light is written in the FBG sensor, and when the light from the broadband source passes through the grating at a particular wavelength, the Bragg wavelength is reflected which is related to the grating period, as shown in Figure 1. The Bragg wavelength can be calculated by equation 1, where eff is the effective index of refraction and Λ is the grating period. Bragg wavelength could be shifted with the variations of temperature, strain, and vibration due to the surrounding environment. The variation of the Bragg wavelength can be obtained by equation 2, where Δ is the strain variation, Δ is the temperature change, is the coefficient of the thermal expansion, is the thermooptic coefficient, and is the strain-optic coefficient.
Figure 1. Working principle of FBG Sensor
(1)
(2)
2.2 Optical Time-Domain Reflectometry (OTDR) Sensors
An OTDR based sensor is capable of distributed sensing over the length of an optical fiber with a specific refractive index (Barnoski et al. 1977). When a light pulse at a particular wavelength propagates along the optical fiber, the sensor can locate the position of the interaction according to the propagation time, as shown in Figure 2. The OTDR-based distributed sensor measures the change in the properties of the light along the entire optical fiber by measuring the time of flight of the returned pulses. The Brillouin optical time domain reflectometer (BOTDR) is one of the well-known distributed fiber optic sensors that is based on the Brillouin scattering. Due to the advantage of being capable of measuring continuous strain and temperature over a long distance, the BOTDR has been widely applied in distributed monitoring of large-scale civil structures.
Figure 2. Working principle of OTDR Sensor
2.3 Fabry-Perot Interferometry (FPI) Sensors
FPI sensors follow the principle of Fabry-Perot interferometry (Yoshino et al. 1982), which is the intensity of the interference signals illuminated between two parallel reflecting interfaces as shown in Figure 3. The interference pattern shifts according to the optical path change and depending on the length of FP cavity. The length can be affected by pressure change and temperature variation (e.g. environmental change). For FPI sensors, the intensity of interference signal is given by equation 2, where I1 and I2 are the reflection at the cavity reflecting interfaces, φ is the initial phase of the interference, Λ is the optical wavelength and Z is the optical path difference (Rao 2006).
Figure 3. Working principle of FPI
Sensor
(3)
3. Applications of Fiber Optic Sensing Technologies for Bridge Health Monitoring
Bridges are critical infrastructures which are vital for daily transportation activities and its health conditions have always been a concern for the bridge owners, contractors and government. Moreover, bridges are subjected to various environmental and operational loadings and continuous real time monitoring is necessary for system identification, damage detection, safety condition assessment, and structural performance prediction. Due to unique and distinctive advantages, fiber optic sensors have been meticulously explored as an effective tool for monitoring various structural components of bridges (decks, towers, stay cables, suspenders, girders, piers, piles, and abutments), for measuring different physical parameters such as strains, temperatures, accelerations, deflections/displacements, cracks, and corrosion detailed in the following sections.
3.1 Comprehensive Bridge Monitoring
Numerous fiber optic based SHM systems have been developed and effectively deployed on various bridges worldwide. Barbosa et al. developed novel weldable strain and temperature sensors based on FBG and installed on circular pedestrian steel bridge for loading tests and evaluate the real time variations of the axial forces in its main structural elements (Barbosa et al. 2008). Mehrani et al. developed a remote sensing system utilizing fiber optic sensors based on FPI for condition assessment of bridge by monitoring its behaviour under traffic loads (Mehrani, Ayoub, and Ayoub 2009). The system was deployed for field instrumentation on a bridge in Florida, USA, during its construction stage. The accuracy of the sensors was estimated by static and dynamic testing under heavy truck loads and validated through 3-dimensional analytical finite element analysis. Kerrouche et al. developed a low cost and compact FBG-based monitoring system incorporating a scanning Fabry Perot filter for strain measurement (Kerrouche et al. 2009). The performance of the system was validated in a field test by installing sensors into CFRP reinforcement rods of a damaged bridge. Rodrigues et al. developed an FBG-based system with embedded displacement and strain transducers for long-term monitoring of structural performance of concrete bridges which was applied to a concrete bridge (Rodrigues et al. 2012). Glisic and Inaudi developed a novel method using distributed fiber optic technology based on Stimulated Brillouin scattering for integrity monitoring of bridges to detect cracks and local deformations (Glisic and Inaudi 2012). The method utilized a glass fiber reinforced thermoplastic composite tape integrated with polyimide-coated optical fibers and deployed on-site by attaching on concrete slab and steel girders of a 1000m long bridge in order to detect and localize cracks and unusual short-term and long-term strain changes. Mokhtar et al. designed a compact temperature compensated FBG based strain sensors sandwiched between two polypropylene slabs and installed on the paragrid and on the surfaces of a flexi-arch bridge to monitor strain and temperature (Mokhtar et al. 2012). Surre et al. developed an optical fiber sensor system based on FBG for long-term strain monitoring and condition assessment of a redundant 50-year-old concrete footbridge (Surre, Sun, and Grattan 2013). Yau et al. developed a simple, inexpensive, and practical method based on FBG strain and tilt sensors to measure vertical displacements of bridges (Yau et al. 2013). An inclination approach and a curvature approach using these sensors was employed to determine vertical displacements with various support conditions and varying stiffness (EI) along the spans of the bridge which is further validated through numerical simulations. Zhao et al. developed multiscale fiber optical sensing network by combining distributed optical fiber sensing technology based on Brillouin scattering and the FBG sensing technology for bridge strain monitoring with local high precision and distributed high precision respectively (Zhao et al. 2012). Minardo et al. demonstrated stimulated Brillouin scattering based distributed FOS for monitoring strain distribution along a 44.4 m long road bridge (Minardo et al. 2012). The optical fibers were installed along the supporting beams and the results yielded a spatial resolution of 3 m and a strain resolution of 15 µε. Xu et al. investigated field monitoring of a 1108 m suspension bridge using integrated distributed FOS based on high resolution Brillouin time domain analysis system with differential pulse-width pair (DPP) technique (Xu et al. 2016). The system monitored distributed strains along the whole length of the bridge with up to 0.05 m spatial resolution, and 0.01 m/point sampling interval.
3.2 Bridge Cables and Suspenders Monitoring
Cables and suspenders are one of the most critical structural components of a bridge that readily suffer from fatigue damage, corrosion damage, and their coupled effects (Lan and Li 2009). Li et al. developed a self-sensing smart stay cable assembled with FBG strain and temperature sensors incorporated into a glass fibre reinforced polymer (GFRP) bar (Li, Ou, and Zhou 2009). The system was inserted into the hollows of steel wires and fixed with the steel cable to monitor deformation, stress and fatigue within the cable. The efficiency of the developed smart stay cable was proved through field application by evaluating the induced stresses and fatigue accumulative damage of a stay cable bridge in China (Li, Zhou, and Ou 2011). He et al. designed a cable force monitoring system by combining local high precision FBG sensors and full scale distributed BOTDR sensing technique in one single optical fiber and installed along with the steel cable wire (He, Zhou, and Jinping 2013). The cable force obtained from the developed sensor agreed well with theoretical value with relative error less than ± 5%. Hu et al. conducted an indirect measurement study for cable force monitoring by developing a FBG vibration sensor for online monitoring of the cable vibration characteristics (Hu et al. 2017). The sensor was deployed on Tongwamen bridge, China and the monitored vibration frequency was converted into cable force according to the theory of string vibration in order to achieve an indirect measurement of bridge cable force.
3.3 Railway Bridge Monitoring
Scott et al. evaluated a FOS system based on commercially available FBG for strain monitoring in railway bridges by conducting laboratory tests under the bridge loading conditions (Scott et al. 2013). The experimental results showed that the FBG strain sensor can obtain good agreement compared with electrical resistance strain gauges. Wei et al. designed a FOS system based on FBG to monitor the bridge strengthening effect accurately by measuring vertical deflection, dynamic load moment and transverse vibration of the railroad bridge (Wei et al. 2013). Wei concluded that the developed system can be extended to large span and arch bridges. Yoon et al. utilized Brillouin optical correlation domain analysis (BOCDA) technology to monitor the longitudinal strain on the rail and girders of a railway bridge during train passage in real time (Yoon et al. 2016). The performance of BOCDA in measuring distributed strain was comparable with conventional strain gauges. Yu et al. developed an SN model of a typical heavy haul railway bridge of China high speed railway and designed a FBG-electrical based multi-sensor for fatigue load monitoring (Yu et al. 2018). The multi-sensor consisted of a fiber-reinforced polymer optical FBG, an electrical resistance strain gauge, a linear variable displacement transducer, and an accelerometer.
4. Conclusions
This paper provides a brief review of various fiber optic sensing technologies employed for structural health monitoring of bridges. Bridges are an integral part of society’s infrastructure and reliable sensing methods are necessary to monitor them and ensure their safety and efficiency. FOS technology has proven to be a suitable method for this purpose and possesses several distinct advantages over other monitoring methods. The simplicity, immunity to EMI and corrosion, distributed long-range sensing, non-invasive sensing (due to the miniature size of FOS), and signal sensitivity of FOS systems enable them to become a popular and effective structural health monitoring approach. However, certain challenges and limitations of FOS such as fiber handling issues, bending losses, cross-sensitivity between thermal strain and mechanical strain exists and still needs to be addressed. In conclusion, FOS have shown a promising potential over other conventional methods in the monitoring of bridge infrastructure.
5. References
Cite This Work
To export a reference to this article please select a referencing stye below:
Related Services
View allDMCA / Removal Request
If you are the original writer of this essay and no longer wish to have your work published on UKEssays.com then please click the following link to email our support team:
Request essay removalRelated Services
Our academic writing and marking services can help you!
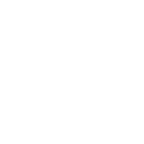
Freelance Writing Jobs
Looking for a flexible role?
Do you have a 2:1 degree or higher?
Study Resources
Free resources to assist you with your university studies!